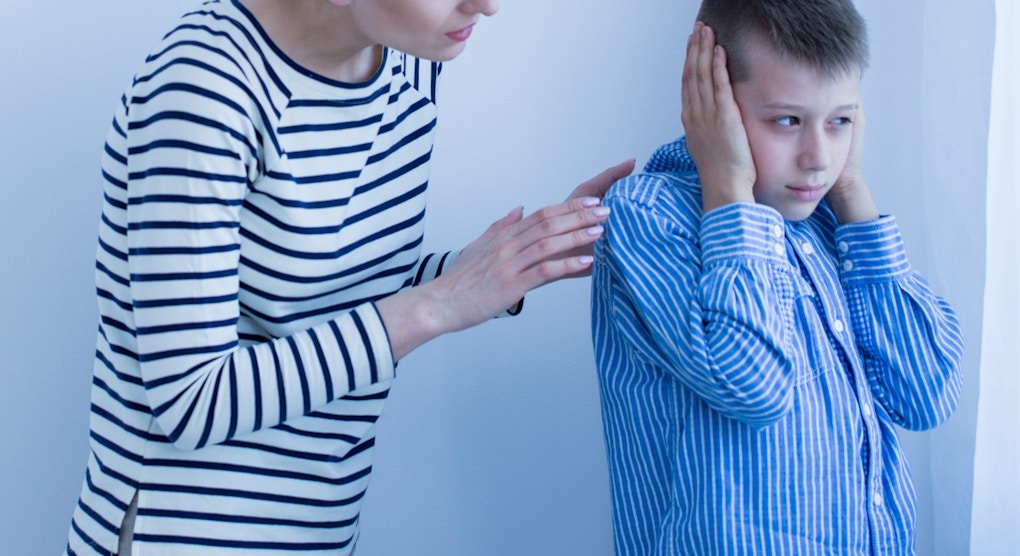
Photographee.eu / Shutterstock
That many individuals with autism spectrum disorder have altered sensory perception has been known since the early reports of the disorder. It was not until recently, however, that abnormal sensory experiences were listed as a diagnostic feature in the Diagnostic and Statistical Manual of Mental Disorders (DSM-5) and they have become an active topic of investigation in the autism research community1.
There are several reasons for this increased research interest. First, because abnormal sensory experiences have been reported as early as six months of age, they could provide a timely developmental marker of the disorder; second, studies have implicated abnormalities in low-level sensory processing in dysfunction in other affected domains in the disorder, such as social cognition and language; lastly, as the neural circuits underlying sensory processing are well studied and relatively conserved across species, investigating altered sensory perception in autism may ultimately help illuminate aspects of the disorder’s neurobiology and identify therapeutic targets.
But despite these advances, many questions remain open. For instance, it is unclear whether atypical sensory processing is a core ASD phenotype or is secondary to impairments in other affected domains (e.g., in social cognition), and the genetic causes — as well as the neurobiological mechanisms that may mediate the link between sensory processing and other key aspects of ASD pathophysiology — are poorly understood.
To discuss these and related questions, SFARI held a workshop on sensory perception in ASD on September 25, 2018. Goals included discussing the status of current research as well as bringing together investigators that conduct animal- and human-based studies to promote cross-fertilization between these traditionally distinct approaches and promote opportunities for translational research.
Spearheaded by SFARI Senior Scientist Brigitta Gundersen, the workshop featured presentations and discussions by several SFARI-funded and non-SFARI-funded scientists. Attendees included Dora Angelaki (New York University), Mark Bear (Massachusetts Institute of Technology), Susan Bookheimer (University of California, Los Angeles), Michela Fagiolini (Harvard Medical School), Jennifer Foss-Feig (Icahn School of Medicine at Mount Sinai), Andreas Frick (INSERM), David Ginty (Harvard Medical School; Howard Hughes Medical Institute), Shulamite Green (University of California, Los Angeles), Lauren Orefice (Harvard Medical School), Carlos Portera-Cailliau (University of California, Los Angeles), Caroline Robertson (Dartmouth College), Susanne Schmid (University of Western Ontario), Noam Sobel (Weizmann Institute of Science), Duje Tadin (University of Rochester) and Mark Wallace (Vanderbilt University).
Martin Raff (University College London), a long-term member of the SFARI scientific advisory board, also attended the event. Raff was among the first who initiated the idea of a workshop on sensory processing in ASD.
Altered sensory and multisensory processing in autism
Altered sensory experiences in ASD may manifest as either hypo- or hyper-sensitivities and affect any sensory modality. Focusing on vision, Duje Tadin described findings from his research on visual motion perception. He demonstrated that neurotypical individuals find it increasingly difficult to identify the direction of movement of visual stimuli (i.e., moving gratings) presented at high contrast, as the size of the gratings increases2. This effect, which is referred to as ‘spatial suppression,’ may be relevant to ASD because it is thought to depend on neural inhibitory processes that have also been implicated in the disorder3. Consistent with the possibility that this mechanism may be altered in ASD, Tadin discussed a study he conducted with Jennifer Foss-Feig, who also attended the workshop, in which they showed that individuals with ASD performed better than neurotypical controls at a spatial suppression task. Specifically, he reported that, although people with ASD found it harder to identify the direction of moving gratings as the gratings become larger, for high-contrast stimuli, they were faster than neurotypical controls at detecting the direction of motion regardless of the size of the gratings4 (Figure 1).

In addition to these findings, Tadin also described a study he recently conducted with SFARI Investigator Scott Murray, where he demonstrated that neurotypical males exhibited shorter visual detection thresholds than neurotypical females in a visual motion detection task5. While examining sex differences at a spatial suppression task in individuals without ASD, Tadin and Murray’s study is relevant in the context of this workshop, not only because the observed results mimic the differences in visual motion perception between individuals with and without ASD described above, but also because ASD has a higher prevalence in males. However, more research is needed to fully understand a potential link between sex- and disorder-based differences in visual motion perception and their role in ASD pathophysiology.
Another sensory modality that was discussed was olfaction. A key form of chemosignalling that modulates many social behaviors of terrestrial mammals, including humans, the sense of smell may be especially important in ASD, which is characterized by difficulties in social functions. Accordingly, Noam Sobel hypothesized that individuals with ASD would show differences in the way they perceive body odors from other individuals and use this sensory information in social contexts. A recent study from his lab found that although individuals with ASD perceived and made judgments about whether an odor was pleasant or unpleasant similarly to neurotypical adults when the odors were detectable (i.e., participants were aware of smelling an odor), differences were observed when the odors were undetectable (i.e., participants were unaware of smelling an odor)6. These results suggest that perception of smells in ASD may differ depending on whether the odors are consciously or unconsciously perceived and, most crucially, that greater impact of this sensory modality on other behavioral domains, such as social interactions, may occur subliminally.
Alongside examining how individual sensory modalities may be affected in ASD, the workshop also discussed whether multisensory integration is altered in the disorder. As the external world constantly bombards the senses with stimuli from different modalities, the capacity to integrate this disparate information into coherent percepts is crucial to form meaningful experiences of reality. Difficulties in multisensory integration may thus have a critical impact on the way life is experienced.
SFARI Investigator Mark Wallace argued that one way the brain achieves multisensory integration is by combining information from different sensory modalities based on the temporal window within which they are perceived7. In individuals with ASD, however, this temporal window appears to be extended relative to neurotypical individuals, thus suggesting abnormalities in the way multisensory integration is achieved8. One interesting aspect of Wallace’s research is that he also found that the boundaries of the temporal window within which different sensory stimuli are perceived can be manipulated by training. This finding opens up the possibility that behavioral interventions targeting this aspect may help restore multisensory integration capacities in individuals with ASD. As this research indicates that temporal binding in ASD is specifically associated with the simultaneous perception of visual and auditory stimuli in a linguistic context, it would be important to investigate the impact of multi-modal integration and potential interventions on the sensory temporal binding window on speech and language deficits in ASD.
Neurobiology of sensory perception in ASD
One way to elucidate the neurobiological bases of abnormal sensory responses in ASD is to use neuroimaging techniques to visualize differences in brain activity associated with perceiving sensory stimuli between neurotypical individuals and individuals with ASD. Shulamite Green, who uses this approach, discussed findings from her research with Susan Bookheimer, also at the workshop, on the brain circuits associated with ‘sensory over-responsivity,’ an enhanced reaction to sensory stimuli that is often associated with negative emotions in ASD. In her studies, Green reproduced the unpleasant quality of those sensory perceptions by presenting participants, while lying in the scanner, with mildly aversive sensory stimuli, such as white noise, rotating color wheels or a scratchy fabric rubbed against the skin. Results from these studies showed that, relative to controls, individuals with ASD showed greater brain activation in primary, association and limbic cortices, as well as subcortical structures for emotional processing9,10 (Figure 2). In addition to describing potential neural substrates of over-responsivity in ASD, she also proposed a mechanistic explanation of these findings, which would suggest that greater brain activation in ASD reflects failure to habituate to the presented stimuli. In other words, individuals with the disorder may overrespond because they treat familiar stimuli as if those stimuli were new10.
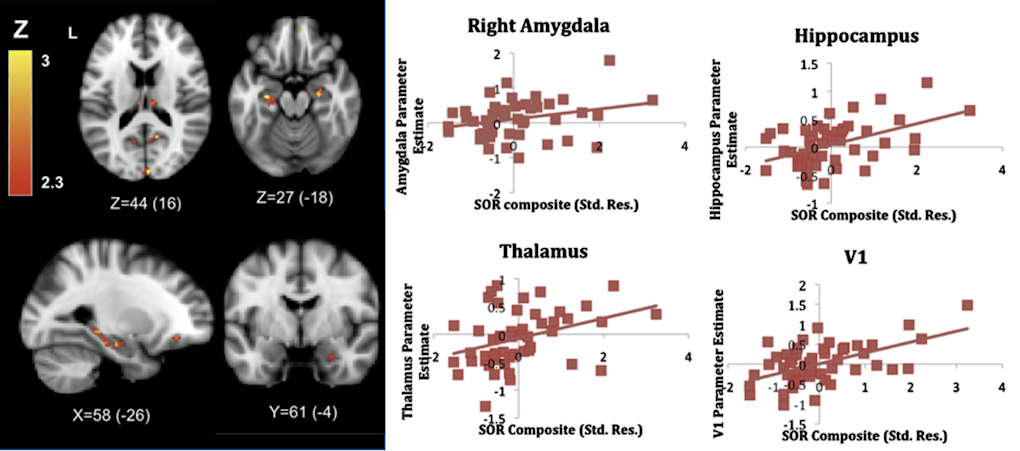
While neuroimaging studies are critical to identify brain-behavior correlates in humans, a deeper understanding of the cellular and molecular mechanisms of atypical sensory processing in ASD is most likely to come from studies using animal models. One such study was presented by SFARI Investigator David Ginty and his former postdoctoral fellow Lauren Orefice, now at Massachusetts General Hospital and Harvard Medical School. Ginty and Orefice examined the somatosensory transduction pathway in several mouse models carrying mutations in autism-risk genes, including Mecp2, Gabrb3, Shank3 and Fmr1. They found that these diverse genetic models exhibited hypersensitivity to light touch. Furthermore, deletion of Mecp2 or Gabrb3 exclusively in peripheral somatosensory neurons, and not neurons in the brain, affected presynaptic inhibition of sensory signal transmission to the spinal cord and ultimately altered tactile sensitivity11.

AdvillinCreERT2; Gabrb3f/+) showed preference for a novel mouse relative to an empty cup, similar to controls. Figure from Orefice L. et al.11.
They also reported that there is a developmental requirement for normal sensory input for acquisition of normal behaviors in adulthood, as deletion of Mecp2 and Gabrb3 only in peripheral somatosensory neurons at early stages of development was associated with behavioral deficits not only in tactile perception, but also in the social and anxiety domains (Figure 3). Conversely, restoring Mecp2 expression in peripheral mechanosensory neurons in mice that otherwise lacked this gene was sufficient to restore not only tactile sensitivity, but also anxiety and social behavior to control levels. These findings suggest that the mechanisms underlying abnormal sensory processing in ASD may involve neurons outside the central nervous system and, similar to what it is observed in humans, lend credence to the possibility of a neurobiologically mediated link between altered low-level sensory processing and emotional and social behaviors impairments in ASD. Ginty was awarded a SFARI Research Award to build on these findings and to begin investigating potential forms of treatment that target these pathways.
Hypersensitivity to sensory stimuli was also discussed by SFARI Investigator Andreas Frick, who presented his latest findings on the cellular mechanisms associated with abnormal tactile processing in an Fmr1 mouse model of fragile X syndrome, one of the leading genetic causes of ASD. He showed that, relative to control mice, Fmr1 mutant mice showed increased neural excitation in the somatosensory cortex when their whiskers were touched. Looking deeper at the underlying cellular physiology, he found dendritic hyperexcitability that was associated with the dysfunction of ion channels, including the BK calcium channel12. These findings suggest that alterations in these channels may provide a key cellular phenotype underlying hypersensitivity in ASD. Frick was recently awarded a SFARI Pilot Award to continue his investigation of these channels and to expand his studies to other sensory modalities and additional ASD mouse models. Preliminary results from these studies suggest that sensory information processing is rather more nuanced than previously thought, in line with human clinical findings.
Translational studies
Because the neurobiological circuits underlying the sensory system are relatively conserved across species, studying sensory processing in ASD may offer opportunities for translational research and developing behavioral and neural markers of the disorder. A key goal of the workshop was therefore to bring together human and animal researchers to facilitate discussion about cross-species approaches to studying sensory processing in ASD and potentially identifying biomarkers.
A proponent of employing a comparative approach to investigating sensory processes in ASD is SFARI Investigator Caroline Robertson. In her presentation, she argued that one limitation in ASD research is the lack of experimental paradigms that can be adapted to both animal- and human-based research. To help bridge this gap, she studies low-level visual-processing mechanisms by using tasks in humans that are amenable to translation in animal studies. One such task, ‘binocularly rivalry,’ refers to the process whereby, when two images are presented simultaneously, one to each eye, the visual cortex sees only one image at a time. Because the brain processes one image while suppressing the other, the time an individual spends seeing each image may thus provide a behavioral measure of the underlying interplay of neural excitation and inhibition. As an imbalance in neural excitation and inhibition has been suggested as one possible mechanism underlying ASD3, this task may provide a gateway to study ASD phenotypes. Robertson had previously discussed her studies on binocular rivalry in individuals with ASD13,14 at a SFARI workshop earlier in the year. At the current workshop, she emphasized the potential of this paradigm to be adapted to animal studies. In fact, she now plans to extend her human research studies by examining binocular rivalry in genetic models of ASD through a collaboration with SFARI Investigator Mark Bear.
Bear, who also attended the workshop, similarly discussed the importance of identifying experimental paradigms that can be used in both animal and human research studies. To this end, he provided the example of a behavioral marker called the ‘vidget.’ The vidget (i.e., a visually induced fidget) is a spontaneous forepaw movement that head-fixed mice produced when they were shown a novel stimulus in a visual memory recognition task15. He argued that vidget habituation, which is thought to constitute a behavioral correlate of plasticity in the primary visual cortex, may be similarly observed as changes in gaze frequency in neurotypical adults performing a similar task. Leveraging the similarities between the mouse vidget and the human gaze response, Bear is now collaborating with SFARI Investigator Charles Nelson to optimize these paradigms for use in studies with ASD mouse models and human participants. A SFARI Research Award is supporting their work.
Speakers at the workshop also emphasized the fact that better communication between animal and human researchers may stem from scientific events where scientists using different research approaches can meet fellow researchers and discuss the challenges and opportunities of conducting cross-species studies. Accordingly, SFARI Investigator Carlos Portera-Cailliau recounted how his collaboration with SFARI Investigator Craig Erickson started thanks to a previous SFARI Investigator meeting, where he proposed the idea of using analogous behavioral tasks in mice and humans. One outcome of this partnership is a recently published paper, where Portera-Cailliau and Erickson used a similar version of the same experimental paradigm to test visual discrimination in Fmr1 knockout mice and individuals with fragile X syndrome; comparable impairments were observed in both species16 (Figure 4). As research using mouse models can further the understanding of the underlying cellular deficits, the possibility of establishing a comparative behavioral outcome measure provides a crucial step toward pinpointing the neural mechanism and circuits affected in humans.
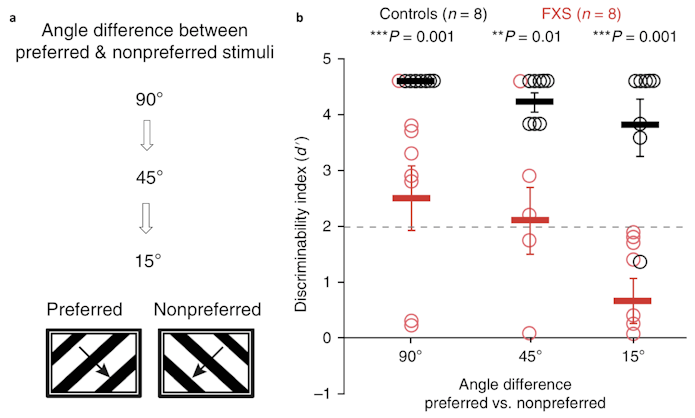
While most translational studies discussed at the workshop focused on the visual system, other sensory modalities may also offer opportunities to conduct cross-species research. For instance, SFARI Investigator Susanne Schmid described her research on auditory perception. In particular, she discussed her plans to establish a translational behavioral platform to probe implicit and perceptual responses to sound and to audiovisual stimuli by using the same paradigms in rat models and children with ASD. This project, which is funded by a SFARI Explorer Award, will use a genetic rat model of ASD that carries homozygous loss-of-function mutations in Cntnap2. In a recent study, she showed that, relative to the wildtype, this model system exhibits increased acoustic reactivity, as well as changes in auditory habituation and prepulse inhibition17. Since the auditory brainstem responses were reduced, she hypothesized that this higher reactivity to sounds might be caused by compensatory mechanisms in response to reduced sensitivity, rather than indicating increased sensitivity. In fact, preliminary results suggest that similar compensatory mechanisms occur at the cortical level, leading to an increased perception of sound.
Suggestions and recommendations
The workshop highlighted the opportunities and challenges of studying altered sensory perceptions in ASD. A lower-level aspect of behavior that is a key component of ASD pathophysiology, atypical sensory processing may provide opportunities to investigate not only its core perceptual aspects, but also its relationship with other functions in the emotional, social and cognitive domains. Moreover, because of the complex nature of the disorder, focusing on sensory alterations, which are most likely to be found in animal models, also provides opportunities for translational research.
A number of challenges, and suggestions, were also considered. SFARI Investigator Susan Bookheimer remarked that, while comparative research may be crucial to identifying biomarkers that can serve as objective measures of ASD pathology, as well as for quantifying change after intervention treatments, there are differences between animal- and human-based research approaches that need to be taken into account. For instance, she stressed that, regardless of how comparable experimental paradigms may appear on the surface, rodent models are often raised in deprived environments. This is in contrast to humans, who develop and live in enriched, noisy, highly stimulating environments. Then, she argued that, if treatment interventions are to be developed for infants, research on sensory biomarkers should focus especially at the early stages of sensory development. Lastly, she reminded the group that questions of biological convergence (i.e., that different genes may affect the same pathways) ought not to be dismissed.
Another SFARI Investigator, Dora Angelaki, emphasized the importance of considering not only sensory processing as a bottom-up process, but also a top-down one, where factors such as inference and context play a role in modulating perceptual experience. She hypothesized that individuals with ASD may present with alterations in nonlinear brain computations underlying multisensory integration, such as casual inference18, and argued that more computational approaches are needed to study sensory perception in ASD. While the neurobiological underpinning of these computations is not yet well understood, theoretical frameworks may help account for some of the impairments that were discussed by animal and clinical researchers.
The workshop ended on a collaborative note. Synthesizing many of the topics discussed during the day, SFARI Investigator Michela Fagiolini acknowledged that providing answers to the questions raised by the workshop is a task no single laboratory could do in a lifetime. For this reason, she called for more collaborations between labs and institutions, and initiated a conversation about joining efforts in a more structured way, for instance, by establishing a sensory consortium. Such a consortium would provide an opportunity for the field to set standards for testing, ensure reproducibility of results and to increase the portfolio of analyses across a wide population of ASD individuals.
SFARI plans to continue supporting research on sensory processing in ASD and to explore how it can facilitate collaborations between researchers working with animals and humans.
References
- Robertson C.E. and Baron-Cohen S. Nat. Rev. Neurosci. 18, 671-684 (2017) PubMed
- Tadin D. et al. Nature 424, 312-315 (2003) PubMed
- Rubenstein J.L. and Merzenich M.M. Genes Brain Behav. 2, 255-267 (2003) PubMed
- Foss-Feig J.H. et al. Nat. Neurosci. 33, 8243-8249 (2013) PubMed
- Murray S.O. et al. Curr. Biol. 28, 2794-2799 (2018) PubMed
- Endevelt-Shapira Y. et al. Nat. Neurosci. 21, 111-119 (2018) PubMed
- Powers A.R. et al. J. Neurosci. 29, 12265-12274 (2009) PubMed
- Siemann J.K. et al. Front. Behav. Neurosci. 8, 456 (2015) PubMed
- Green S.A. et al. J. Am. Acad. Child Adolesc. Psychiatry 52, 1158-1172 (2013) PubMed
- Green S.A. et al. JAMA Psychiatry 72, 778-786 (2015) PubMed
- Orefice L.L. et al. Cell 166, 299-313 (2016) PubMed
- Zhang Y. et al. Nat. Neurosci.17, 1701-1709 (2014) PubMed
- Robertson C.E. et al. Curr. Biol. 26, 80-85 (2016) PubMed
- Robertson C.E. et al. J. Neurosci. 33, 16983-16991 (2013) PubMed
- Cooke S.F. et al. Nat. Neurosci. 18, 262-271 (2015) PubMed
- Goel A. et al. Nat. Neurosci. 21, 1404-1411 (2018) PubMed
- Scott K.E. et al. J. Neurosci. 38, 8588-8604 (2018) PubMed
- Rosenberg A. et al. Proc. Natl. Acad. Sci. USA 112, 9158-9165 (2015) PubMed