
Antoine Taccetti/Getty Images
**Note: The SFARI Autism Rat Models Consortium request for applications is opening early July 2021.
Workshop highlights
- Rats are a highly trainable species, displaying robust and reliable behavior; yet they also express a variety of innate, complex behaviors, including a rich social repertoire.
- The future of systems and behavioral neuroscience should integrate data-rich, automated phenotyping technologies with ethological expertise.
- The field would benefit from an interpretative framework that incorporates computational perspectives with a variety of experimental strategies, from experimenter-defined tasks to naturalistic behaviors.
- To reveal the multitude of developmental and compensatory changes that occur in autism, it will be important to use the rat’s complex behavior to sufficiently challenge the system and reveal the underlying circuit biology.
- Cross-species research, whether it be comparing the rat to other model systems or to the human, will be vital to future translational impact.
- Such an ambitious research agenda will likely require coordination across multiple labs, possibly through a consortium.
Hundreds of genes have now been linked to autism risk. To understand how these genetic changes affect biology and behavior, researchers have been manipulating autism risk genes in diverse model systems, spanning from fruit flies, through worms, to mammals. Among the mammalian models, mice have historically been the most widely used, largely because of their ease of genome manipulation. However, as new genome editing techniques are becoming more refined, disease-associated gene mutations can now be readily applied to numerous other species; this raises the question of what advantages rodent (and mammalian) models other than mice may afford to study conditions such as autism spectrum disorder (ASD).
On April 26 and 29, 2021, the Simons Foundation Autism Research Initiative (SFARI) and the Simons Initiative for the Developing Brain (SIDB) hosted a virtual workshop that focused on the use of rats as a model system for ASD. Organized by Alice Luo Clayton of SFARI and Peter Kind at SIDB of the University of Edinburgh, the workshop convened over 30 attendees from multiple time zones. The workshop sought to discuss the types of studies that might best exploit the advantages afforded by rat models, specify scientific priorities, assess the need for cross-species validation and evaluate next steps, such as how to best coordinate research efforts across labs.
As Luo Clayton pointed out, “rats are not just large mice.” Rats and mice are species with different adaptations and have separately evolved for the past 12 million years. Rats evolved in very different ecologies from mice and are an innately social species that lives in large cooperative groups. Kind noted some additional characteristics that make rats particularly attractive for behavioral and circuit neuroscience experiments in autism research, including their complex social repertoire, cognitive flexibility and ease of training. Their larger body and brain size can also support recording, stimulating and imaging devices, and their large litter sizes promote within-litter, cross-genotype comparisons.
“All models are wrong, but some are useful,” said Luo Clayton, adapting George Box’s aphorism in statistics to acknowledge that no one animal, cell or computational model would ever recapitulate human autism. “With this workshop, we wanted to bring together some of the best scientists to help us think about how rats can be useful to address unanswered questions in autism research and to learn how SFARI and SIDB can build upon the resources we have already developed.”
Behavioral phenotyping: Integrating technology with ethology
A major challenge that animal researchers face is how to analyze and interpret behavior, especially if behaviors are complex and spontaneously occurring. Bence Ölveczky of Harvard University and Bob Datta of Harvard Medical School argued that technology may help capture and interpret phenotypes.
Ölveczky has worked on several approaches for tracking 3-D movement in freely behaving rats. One system combines marker-based motion capture and deep learning to collect information about joint position over time, then extracts from that specific behavioral components, such as rearing-up, locomotion and grooming1. The system detects differences in Fmr1 knockout rat behavior, like increases in grooming bout lengths, as well as novel, idiosyncratic grooming sequences not seen in wildtype. Ölveczky also described a marker-less system, called DANNCE (3-Dimensional Aligned Neural Network for Computational Ethology), that uses anatomical landmarks and a convolutional neural network to estimate 3-D positions2 (Movie 1). To train the network required a lot of data—seven million time points—but DANNCE can be applied to rat pups, to other species like mice or marmosets and to social interactions between rats.
Datta described his efforts to discover the underlying structure of behavior through Motion Sequencing (“MoSeq”), which combines 3-D imaging and machine learning to identify units of behavior, or “syllables”3. In mice, MoSeq finds 40–60 motion syllables, which include examples named “move it along,” “down and dart” and “scrunch.” The syllables can be put together in a variety of sequences that ultimately culminate in behavior. These behavioral units seem to be biologically meaningful, in that neural activity in the striatum aligns with transitions between syllables. MoSeq can extract syllables even when faced with behavioral diversity elicited by psychoactive drugs, and it can also predict which drug a mouse received based on its behavior. Datta is now applying the technique to autism-mouse models, rats and social situations. A current limitation of MoSeq is that it is focused on a single timescale, so future work could try to track interactions between multiple animals across multiple timescales.
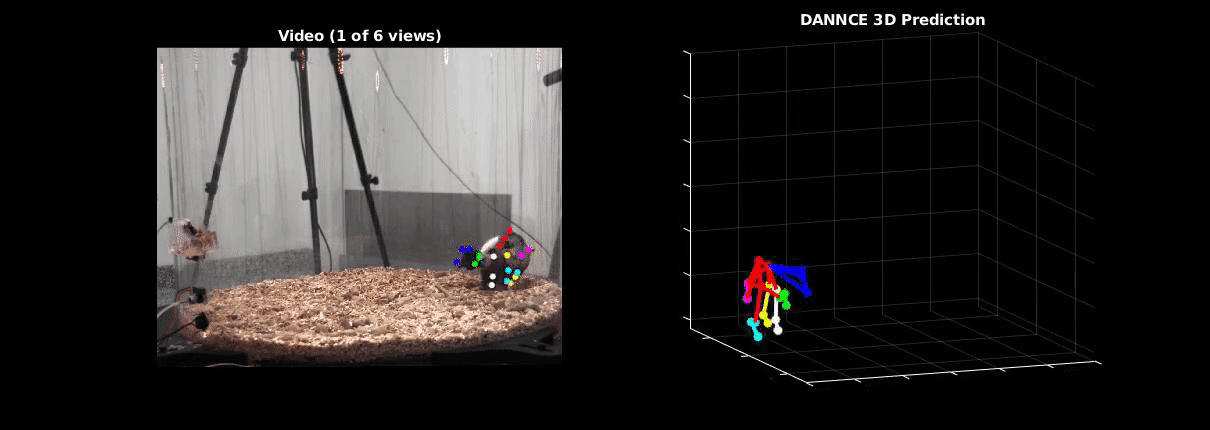
During discussion, Ölveczky and Datta agreed that acquisition of high-resolution behavior is a solvable problem in the near future, but making sense of that data will be a much harder challenge for the field. This could be mitigated, in part, by getting more computer scientists into neurobiology. While the hardware needed to run these systems are not prohibitive, the analysis of the data from automated systems is complex, making it difficult for many investigators to add to their research portfolio. Datta received a federal grant to make MoSeq more accessible to other labs. Funding specifically set aside for such platform dissemination will be critical to the democratization of systems like MoSeq and DANNCE.
Mriganka Sur of the Massachusetts Institute of Technology, noted that large amounts of data are required to dissect the modular components of behavior, and understanding the rules for stringing them together will be important for understanding not just autism, but all behavior. The adoption of more unbiased, automated methodologies could bring some helpful standardization to analysis of naturalistic behaviors.
Yet others reiterated the need to maintain creative thought from trained behavioral scientists to ensure the detection of novel behaviors. Illustrating this point, Oliver Hardt of the University of Edinburgh recounted a case study with Nlgn3 knockout rats. In a standard fear-conditioning task, the knockout rats froze less than the wildtype rats in response to a shock-predicting tone, which is typically interpreted as a deficit in fear learning or fear memory. But the researchers also noted that the knockout animals could not be trained in a different fear-learning task because most of them jumped out of the apparatus – something wildtype rats rarely, if ever, do. This prompted additional careful behavioral analyses, revealing that fear learning indeed was intact in the knockout rats, but that loss of Nlgn3 caused a bias towards flight behavior rather than freezing, compared to the wildtype rats. Subsequent experiments provided evidence that altered cellular excitability in the periaqueductal grey mediated this bias. This story emphasizes the need to consider eco-ethological perspectives when interpreting animal behaviors as animals have a wide variety of options to respond in each situation. Standard laboratory tasks suggest that animals should behave in a certain way and that behavioral differences indicate a task-specific performance deficit. Yet, these differences may reveal that animals interpreted the situation differently and responded accordingly, i.e., they are capable of expressing the behavior normally elicited in the task, but they decide to do something else.
Ensuring an ethological perspective in rat-based research is also a priority of SIDB, where researchers have developed the ‘Habitat’ (Figure 1), a multi-story modular environment reminiscent of the underground burrows that rats inhabit in the wild. This naturalistic environment has the potential to uncover differences in social behaviors, such as those involved in dominance, and reveal novel behavioral phenotypes not possible under typical animal housing conditions. All the rat models created with SFARI support and phenotyped at SIDB will be also examined in the Habitat.
Jill Silverman of the University of California, Davis has studied a number of rat models of autism, and noted several advantages over mice, including reduced behavioral variability, larger body sizes that ease handling of young pups for developmental studies, as well as innate social behavior, such as social communication. Acknowledging that a reliance on rodent behavior has been blamed for much of the failure of pre-clinical insights to translate to human clinical trials, she advocated for quantitative behavioral readouts and more attention to genetic background and breeding protocols to improve reproducibility.
Rat’s cognitive abilities and behavioral flexibility allow for complex tasks
Rats express a wide variety of complex, innate behaviors, which can be leveraged in various experimental approaches to understand the underlying biology.
In his talk, Loren Frank of the University of California, San Francisco, focused on spatial cognition, a behavior well-studied in rats that could be useful for uncovering differences in the cognitive domain associated with autism. He introduced the M-track spatial alternation task, a complex experimental setup that he developed to probe spatial sequence learning in rats; he argued that complex tasks may have a better chance at revealing deficits that are not apparent in simple paradigms. In a version of the spatial alternation task, Frank reported some preliminary evidence of a learning deficit in Scn2a+/- rats, but not in SFARI-generated Fmr1-/y, a model for fragile X syndrome.
Frank also stressed that computational modeling of complex behavior will be essential for fully understanding how the brain learns. For example, modeling rat performance on a spatial sequencing task revealed that memory alone cannot account for it; the brain must involve other cognitive processes such as spatial bias4. Testing behavior against computational models may provide a mathematical, interpretative framework that helps researchers compare data across species and disciplines. “Without it,” Frank stated, “we are just using words, and we may not all agree on what those words mean.” David Kastner of the University of California, San Francisco, who conducted many of the experiments with the Fmr1-/y and Scn2a+/- rats in the Frank lab, further explained that a computational framework combined with the rat’s expansive behavioral repertoire allows investigators to home in on the features that differentiate the cognitive algorithms used by wildtype and mutant rats.
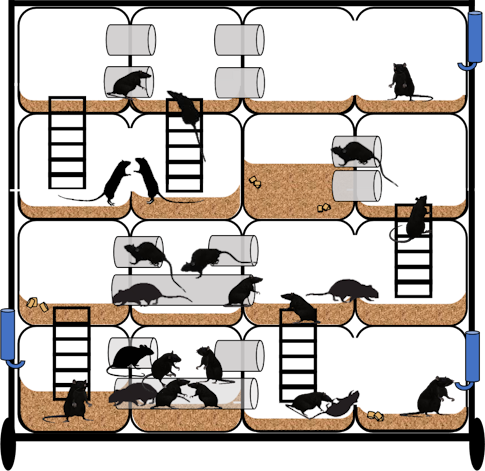
Emma Wood of the University of Edinburgh talked about spatial cognition in the SIDB-generated Fmr1-/y rats developed at SIDB. It takes a complicated task to reveal a deficit. While these rats can detect a novel object in a standard object-recognition task, they show a robust deficit in a task that associates object, location and context. She suggested that overly rigid hippocampal activity may contribute to this: CA1 pyramidal neurons recorded from Fmr1 knockout rats did not decrease their firing rates, tighten their place fields or shift their phase-locking to theta oscillations as CA1 neurons do in wildtype rats as they gain more experience with a new environment. In vitro, these cells also show signs of hyperexcitability and decreased synaptic inputs, which may underlie some of the circuit-level anomalies.
Also focusing on SFARI-generated Fmr1 rats, Laura Colgin of the University of Texas at Austin talked about her work on the role of the hippocampus in social memory. She found that in the wildtype rats, place cells in CA2 typically modulate their activity during social interactions, whereas those in CA1 do not5. In Fmr1 knockout rats, however, preliminary data suggest CA2 place cells are not modulated by social odors, although the animals seem able to smell. Colgin is now examining the functional connectivity between CA1 and CA2 and exploring the possibility that changes in coordination of inputs from the entorhinal cortex prevent CA2 from encoding social stimuli. This might reflect cognitive inflexibility, in that the rats are unable to respond to a change in their social environment.
In the discussion, participants voiced support for using complex tasks (also see Gina Turrigiano’s research using a cricket-hunting paradigm below); but also underscored the importance of keeping simple tasks that are well-defined and well-established. A challenge for the field will therefore be to find the appropriate balance in at least two dimensions: simple versus complex tasks; experimenter-defined versus naturalistic tasks. Each task offers relative strengths and weaknesses with regard to ethological validity and neurobiological interpretability.
Biological compensation and homoeostasis
When using complex tasks, a key question arises: how is the rat’s complex behavior specifically useful for autism research? Sur challenged the group to consider this question. One advantage may be in the context of biological compensation. As Ölveczky noted, the simpler the behavior, the more ways there are to solve it. By layering on complexity, the number of pathways to the solution is reduced, thus uncovering nuanced but meaningful differences.
Developmental processes may also contribute to reallocate certain functions to other circuits. For example, it is striking that the Fmr1 knockouts show such minor phenotypes given the large number of mRNAs regulated by FMRP, the protein encoded by Fmr16. This finding may mean that the brain has found a way to compensate, possibly using other circuitries to get by. If true, knowing what these alternative circuits are would have implications for therapeutic targets and strategies. Peter Kind noted that this compensation could help explain why the many phenotypes at the level of cellular physiology result in modest, often highly variable behavioral phenotypes in Fmr1-/y animal models. Adrian Bird of the University of Edinburgh raised the possibility that compensatory changes may drive autism phenotypes, such as when influenza symptoms are caused by the body’s reaction to the virus, not the virus itself. It is possible that autism, or even some convergence within autism, may be common responses to different perturbations in brain function.
One way to tackle these questions is to focus on the role of homeostatic mechanisms, which help neural circuits maintain stability in the face of plasticity. In her talk, Gina Turrigiano showed how homeostasis is revealed when a system is perturbed. For example, rat visual cortex neurons have stable firing rates that fluctuate around a set point. When visual input is perturbed, for example, by closing an eye, firing rates initially drop off, but eventually recover to their original rates7. Disrupted homeostatic mechanisms may play a part of autism: in dissociated cell cultures made from rat visual cortex neurons, knocking down autism-related gene Shank3 prevented the homeostatic reactions of synaptic scaling and changes in intrinsic firing. Also, in vivo, changing the sensory drive to visual cortex by closing one eye in Shank3 knockout mice caused an expected drop of the firing rate in visual cortex neurons, but one that didn’t recover as in wildtype8.
To further explore homeostasis in the context of complex behaviors, Turrigiano is now exploring cricket hunting as a way to perturb visual cortex activity by tapping into the rat’s instinctive behavior. Rats are intrinsically motivated to chase down and consume a cricket introduced to their chamber. This involves learning, which is initially dependent on vision and on visual cortex activity. SIDB is also using a cricket-hunting paradigm, which will be added to their behavioral pipeline (Figure 2).
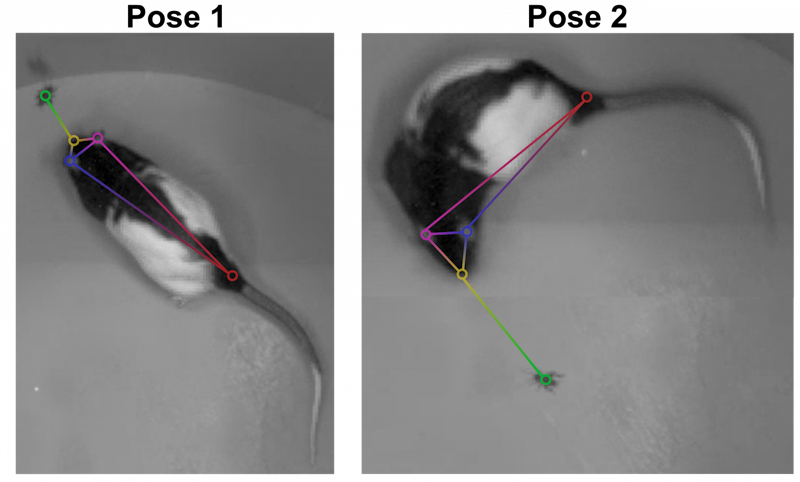
Development
One advantage of using rats instead of mice is that the larger size of rat pups makes it easier to study neurophysiological processes in early development. Mark Blumberg of the University of Iowa discussed his work on sleep in early life and its contributions to brain development. One focus has been the twitch movements that are characteristic of REM sleep and occur many thousands of times a day in both infant humans and rats (View clips showing twitching in rat pups here). Unlike awake movements, which occur simultaneously across many muscles, twitches are discrete, smaller in amplitude and localized to a single skeletal muscle. Tracking brain responses to twitches in young rats, he has found that they promote functional coupling between the hippocampus and the red nucleus, a brainstem motor structure9. Sensory feedback from twitches also triggers responses throughout the sensorimotor system and the primary motor cortex10. Blumberg argued that twitches help create an internal representation of the body, upon which later-emerging social and cognitive functions depend. Thus, the disrupted sleep commonly noted in autism may be more than just another symptom of the condition, but rather a critical developmental context within which other features unfold.
Understanding how age influences the systems that work together to support learning would be important for identifying both strengths and weaknesses in animal models of autism and could eventually inform interventions for people with autism, argued Linda Wilbrecht of the University of California, Berkeley. Using computational modeling and a conditional associative learning task, Wilbrecht has been able to dissect working memory from reinforcement learning in human adolescents and adults11. This revealed an increase in working memory associated with puberty and a more protracted increase in reinforcement learning rate with age. Adapting the task to mice, using odors instead of pictures as stimuli, she finds that mice can learn to discriminate but are slower and not as accurate. Wilbrecht is now applying these methods to mice carrying mutations to ASD risk genes like Tsc2 and Shank3b, and she is planning to use these methods to measure how rats may outperform mice. Such reinforcement learning model approaches can be developmentally implemented in a variety of species, including humans, and may be important for understanding how tasks may be solved in different ways, as well as reveal both vulnerabilities and opportunities in autism.
Dan Feldman of the University of California, Berkeley, asked the participants to consider the possible role of disrupted internal models in autism. He noted Blumberg’s study of neonatal sleep twitches as a developmental example of implementing the ‘forward’ model. What would be the consequences of a brain having a poorly developed forward model? Challenges in predictive coding, which has been theorized in autism12, could have implications in a variety of functional domains—from motor coordination to social interactions. Frank noted the critical involvement of predictive coding in many of the experimental paradigms presented at the workshop.
Looking ahead
The workshop participants agreed that rat models may provide useful insight to better understand the biological mechanisms that lead to the behavioral manifestations of autism. But as Bird pointed out, “rats are only one part of the story; it would be naïve to think that there is only one true model for autism.” The various examples from the workshop and beyond hinted at the scientific potential for cross-species studies. In fact, Sur explained, “each animal model may be a goal in itself,” and the behavioral outcomes will depend on the animal’s biological “manifold” within which a gene change occurs. Systematically tracking the “shifts” across the manifold, in multiple species, may lead to an understanding of the shifts that occur in humans with autism.
But an important question to consider, which was posed by Peter Scheiffele of the University of Basel, is: how much of what has been research-enabling in the mouse should be recapitulated in the rat? A key advantage of mice has been the extensive development of technologies such as Cre-lines, which have been transformative in understanding circuit neurobiology. Should the field try to recreate all of these ‘tool’ lines for rats, or should researchers only use global, constitutive models which are more akin to the human condition?
Participants agreed that the choice of model should depend on the scientific question at hand. For example, even if a cell-specific or conditional knockout of a gene doesn’t happen in autism, it can answer specific questions about circuit effects that lead to insights for systems in which the gene is missing from all cells. Scheiffele noted that the sweet spot, for the time being, may be parallel efforts in mice and rats; experiments in mice could be weighted toward using localized, conditional strategies and initial experiments in rats could be weighted toward circuit and behavioral readouts in the global, constitutive models. New tools can and should be generated in rats as the bespoke questions demand, but simply generating them to have a ‘toolbox’ seems unwarranted at this time.
Finally, the workshop underscored the importance of setting and working toward priorities in the light of increasing translational efforts from rodent studies to human clinical trials of neural disorders. Speaking to this point, John Isaac gave his opinion on what animal models of autism should provide to persuade a company (big or small biotech) to take it on for therapeutic development. In his view, a company would want to identify a reliable, pathological feature of autism for which people seek therapeutic intervention that can also be reliably measured in an animal model. This pathological feature should also come with a target, either for a drug or some other therapeutic approach, that when modulated, affects the pathological feature. In the clinic, researchers would work out assays that are sensitive to detect changes to a target, and to come up with clinical endpoints that regulators would accept. This would need a close interchange between animal models and human work.
Recommendations
Given the ambitious research goals put forth by the workshop participants, Luo Clayton charged the group to specify actionable recommendations that would leverage both SFARI’s convening power and its role as a funding organization.
Participants mentioned the need for a centralized database for rat models, with information on their behavioral characterization and availability. Aron Geurts of the Medical College of Wisconsin put forward the idea of an open-source rat model wiki, in which investigators could find summarized descriptions and share their experiences with various lines. This type of centralized accounting and community sharing could serve as a clearinghouse to prioritize future development of select ‘tool’ models, in addition to aggregating the community’s expertise in behavioral analysis.
A research consortium like the International Mouse Phenotyping Consortium might also be useful; the moderate number of SFARI and SIDB rat models so far means organic collaboration between labs can be a productive way to move forward. Continued meetings with vested researchers could lead to parallel/overlapping approaches across several labs. Yann Herault of the French National Centre for Scientific Research (CNRS) noted the impact of the rat models will only come when researchers with slightly different points of view start seeing the same differences in their models. Others mentioned that collaboration with the Simons Foundation Flatiron Institute has been useful for improving spike-sorting algorithms and could help getting tools to measure behavior into labs, or to analyze the high-dimensional datasets coming from the 3-D movement tracking systems. Another type of collaboration is one in which an experimenter goes to the animal model, as in the case of macaque models housed in centers in China. As the autism field continues to accumulate larger datasets from more complex experimental systems, the needs of the research will go beyond individual labs. Ultimately, a hybrid model for rats may work, with a virtual center organizing activities in individual labs.
“It was energizing to see the level of excitement and potential for this field. Based on the workshop outcomes, SFARI will be releasing a request for applications to transform the workshop’s recommendations into actionable efforts,” said Luo Clayton.
“It was an extremely productive workshop,” said Kind. “A general consensus emerged that rats provide an invaluable resource to better understand the biological basis of complex behaviors and the underlying neural circuits. Important questions were articulated that can provide a research framework for the field over the next few years; we now need to coordinate efforts and implement strategies to answer them.”
“SFARI aims to lower barriers to enable researchers to do the best science possible,” added John Spiro, SFARI’s interim director. “We’ll keep discussing how we can provide support to rat-based studies, facilitate collaborations between groups and promote new discoveries.”
References
- Marshall J.D. et al. Neuron 109, 420-437 (2021) PubMed
- Dunn T.W. et al. Nat. Methods 18, 564-573 (2021) PubMed
- Wiltschko A.B. et al. Nat. Neurosci. 23, 1433-1443 (2020) PubMed
- Kastner D.B. et al. J. Neurosci. 40, 7311-7317 (2020) PubMed
- Alexander G. M. et al. Nat. Commun. 7, 103000 (2016) PubMed
- Darnell J.C. and Klann E. Nat. Neurosci. 16, 1530-1536 (2013) PubMed
- Torrado Pacheco A. et al. Proc. Natl. Acad. Sci. USA 116, 18068-18077 (2019) PubMed
- Tatavarty V. et al. Neuron 106, 769-777 (2020) PubMed
- Del Rio-Bermudez C. et al. Curr. Biol. 27, 1413-1424 (2017) PubMed
- Tiriac A. et al. Curr. Biol. 24, 2136-2141 (2014) PubMed
- Master S.L. et al. Dev. Cogn. Neurosci. 41, 1000732 (2020) PubMed
- Cannon J. et al. Autism Res. 14, 604-630 (2021) PubMed