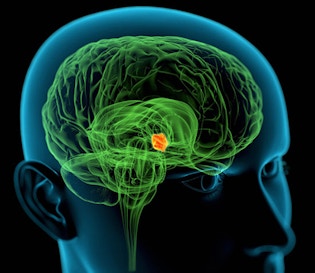
Copyright Photo Researchers, Inc.
Fever when you kiss me, fever when you hold me tight.
Fever in the morning, fever all through the night.
Peggy was on to something.
Even without a hug and a kiss, fever has benefits beyond its role in fighting infection. According to a prospective study published in 20071, and to many anecdotal reports, fever can improve cognitive function and behavior in individuals with autism.
A preliminary survey of families enrolled in the Simons Simplex Collection indicates that as much as 25 percent of parents report some improvement when their children are febrile.
In the prospective survey, the first of its kind, Andrew Zimmerman and his group at the Kennedy Krieger Institute followed 30 children with autism between the ages of 2 and 18 years with fevers above 100.4 degrees Fahrenheit, and a comparable group of children with autism matched for age, gender and language level who were afebrile over the same period of time. Using the Aberrant Behavior Checklist, the researchers documented decreases in hyperactivity, irritability and stereotypy, as well as improved speech during the fever and for a short time thereafter.
More data are needed, but these first reports are so dramatic that it is worth mining fever biology for clues to mechanism and even for potential novel therapeutics.
Thermoregulation:
Temperature is tightly controlled in warm-blooded animals. Neural, endocrine and immune mechanisms are called into play to maintain internal temperature between 98 F and 99 degrees Fahrenheit over a wide range of external temperatures. For example, when core body temperature rises, blood vessels in the skin dilate, and vessels to internal organs constrict in order to dissipate heat.
The temperature set point is not fixed, however. In the face of a bacterial or viral invasion, core temperature rises. When it exceeds 99.5 degrees Fahrenheit, it is called a fever, and a new set point is established at a higher level. Fever combats the infection by activating lymphocytes and other immune mechanisms.
The question before us is how fever or the many compensatory mechanisms might ameliorate signs and symptoms of autism. In principle, one can postulate two mechanisms.
First, the firing of neurons in relevant neural circuits might be enhanced or suppressed by small changes in temperature. Second, neuronal firing might be influenced by circulating chemicals such as prostaglandins (for example, PGE2) or one of the many circulating cytokines (for example, interleukin 6) that are synthesized and released to defend against invading organisms. The two hypotheses are not exclusive. In either case, it is useful to begin with the hypothalamus.
The hypothalamus is a collection of midline nuclei situated deep in the brain adjacent to the third ventricle, at about the level of the eyes. Hypothalamic nuclei receive inputs from brain regions that make up the limbic system, including the amygdala, hippocampus, cingulate gyrus and other regions of the prefrontal cortex. They also receive information from neurons located in the brainstem that regulate autonomic effectors, including the heart, blood vessels, glands, liver and other internal organs.
The hypothalamus talks back to all of the structures from which it receives input. In addition, it sends axons to the pituitary, the master gland that coordinates hormonal responses to stress. Thus, it is in a position to encode and decode hormonal, emotional and cognitive stimuli, as well as autonomic information. This is what makes the hypothalamus so relevant to autism.
Neurons in the hypothalamus, particularly those in the ‘febrigenic’ preoptic nucleus, are sensitive to small shifts in temperature. A change in firing rate might enhance the release of oxytocin, vasopressin and melanocortin from hypothalamic neurons. These peptides have been implicated in social behaviors and responses to stress. Beyond intra-hypothalamic effects, a change in local neuronal firing may change the function of distant cortical circuits that are synaptically connected to the hypothalamus.
The brain, in the main, is protected from proteins and other hydrophilic molecules circulating in the blood. However, the blood-brain barrier at the base of the brain in close proximity to the hypothalamus is relatively porous, so large molecules including cytokines can enter the hypothalamus with relative ease.
The hypothalamus has been implicated in studies of Rett syndrome2, another pervasive developmental disorder. Children with Rett syndrome exhibit sleep disorders and episodes of increased anxiety, along with cardiac, gastrointestinal and respiratory dysfunction — all signs of autonomic dysfunction. Selective deletion of MeCP2, the gene implicated in more than 95 percent of individuals with the syndrome, from a subset of neurons in the mouse hypothalamus mimics many of the effects of deleting MeCP2 from the entire mouse brain.
The hypothalamus is also called to mind by studies that document an increase in the incidence of neuropsychiatric disorders, including autism and schizophrenia, following hurricanes, floods and famines3,4. Such stressors might increase the incidence of de novo germline mutations.
Microcircuits within the hypothalamus are complex and deserve a separate discussion. Whether the trigger is temperature or chemical activation, one must ask about downstream events. Eventually, axons exit the hypothalamus to innervate the cerebral cortex, the amygdala and neurons in the brainstem, including the noradrenergic neurons in the locus ceruleus (LC).
The locus ceruleus: a role in autism?
Last year, Mark Mehler and Dominick Purpura summarized arguments supporting a role for noradrenaline-containing neurons in the LC as an important link between fever and autism5. Chemical ablation of noradrenergic nerve cell bodies in the rodent LC or in the hypothalamus blocks the febrigenic effects of injected bacterial extracts.
There are only 40,000 noradrenergic neurons in the LC, but each of them extends axons that innervate a wide area of the cerebrum, and each axon exhibits many thousands of noradrenaline-filled varicosities along its course that are presumed sites of its release. This wide influence makes LC neurons ideally situated to influence the full complex of symptoms that define the autism spectrum.
Dom Purpura has been thinking about this for some time, as indicated by the following quote from his paper published 30 years ago6:
The occurrence of autistic behavior in a wide variety of neurologic disorders suggests dysfunction of a common anatomic pathway, although the mechanism and severity of the pathologic change may vary.
Gary Aston-Jones and Jonathan Cohen have developed an interesting conceptual framework regarding the LC and aspects of cognition related to arousal and decision-making7. In their scheme, tonic discharge of LC neurons shifts the level of arousal along an inverted U-shaped curve.
At the extremes, cognitive function is compromised. At low levels of LC activity, the cortex is essentially asleep, whereas at high levels, arousal is so extreme that it is impossible to focus on the task at hand. At the midpoint, the level of arousal is optimal, and one can rivet attention on salient environmental cues or rapidly disengage and shift attention between tasks as situations change.
This modern interpretation of the 1908 Yerkes-Dodson Law— which first described this inverted U-shaped curve — would facilitate appropriate decisions in cognitively demanding situations. One bonus of this model is that it doesn’t matter if the output from the hypothalamus during fever is excitatory or inhibitory to the LC, as long as it changes LC activity.
Direct recordings from LC neurons in awake, behaving, non-human primates have shown that periods of tonic discharge are interrupted by brief bursts of activity8. During the optimal, mid-range tonic discharge, the phasic bursts of LC activity correspond, in time, to decisions regarding the task at hand rather than to perception of the task or to reward following the task. This important distinction has implications for our thinking about cognitive and behavioral challenges faced by individuals with autism.
Aston-Jones and Cohen emphasized the ability to rapidly switch between thoughts and behaviors in the midst of a confusing environment. Others have described ‘flips’ in invertebrate behavior patterns.
Two features of the noradrenergic innervation of the cerebral cortex fit well with this scheme. Tonic noradrenergic action is surely influenced by the fact that noradrenaline release sites are not immediately opposed to postsynaptic structures. Rather, noradrenaline packaged in axonal varicosities acts on receptors some distance away from the site of release.
Such paracrine action is reminiscent of hormones and other modulators that act in a sustained, tonic manner. Phasic bursts of activity within the LC also fit with this scheme. Such bursts are amplified by the fact that many LC neurons are electrically coupled to one another (via dendritic gap junctions). Ensembles of neurons, rather than individual cells, fire in synchrony. Such synchronized bursts may underlie the needed rapid modulation in the cortex. A picture emerges of noradrenaline acting as a coarse gain control interrupted by transient increases in output.
It is tempting to think of noradrenaline as the engine of change in the behaviors described above. How might that come about? Noradrenaline can modulate ion channels in smooth, cardiac and skeletal muscle, in autonomic and sensory ganglia, and in CNS neurons. Changes in voltage-gated calcium currents are prominent, but changes in potassium and sodium channels have been described as well. Each of these events might alter emergent patterns of neuronal firing.
It is also well known that noradrenaline plays important roles in early development to influence phenomena such as the proliferation of neuronal precursors, axon outgrowth, synapse formation and neuronal survival. Any or all of these events might contribute to the autism phenotype in the first place, and reversal of any one of them might lead to relief on a longer time scale.
Next steps:
Several issues should be addressed in the near future. More documentation of improvement with fever is needed, perhaps on the scale of an epidemiological survey conducted by the Interactive Autism Network. Quantitative, standardized measurements of fever and rigorous assays of behavior in febrile children with autism should be pursued. A signature neural correlate of improvement revealed by magnetic encephalography or functional magnetic resonance imaging would provide a welcome additional measure.
It is not too early to think about therapeutic interventions. I have focused on noradrenaline in this column. Adrenergic agonists and other stimulants have been useful in modulating aggressive behaviors in some children with autism. One must consider the diversity of adrenergic receptors, transporters and metabolizing enzymes, as well as the heterogeneity of the autism phenotype, in future clinical trials.
Other approaches could also be explored. Clinical trials of oxytocin are already under way, and studies of other hypothalamic peptides should be considered. Agents that interfere with or enhance the action of particular cytokines have not been explored in neuropsychiatric disorders. Interleukin 6 is perhaps the most likely target among these, but there are approximately 100 cytokine genes described to date.
A careful review of prior studies and novel analyses of interacting molecular networks are in order. Cytokine functions overlap, and knockout mice appear normal under resting conditions. It has been argued, therefore, that many cytokines do not play a role in resting physiological states. It may be that the challenge of a fever will uncover unique functions and targets in the brain.
Perhaps most controversial is a call for experimental interventions designed to increase hypothalamic temperature in children with autism and in healthy controls.
In any case, the remarkable success in treating a mouse model of Rett syndrome9 by reversing repression of the MeCP2 gene raises the possibility that the consequences of developmental miscues can be reversed in adult individuals. That is encouraging.
Prescriptions in such a broad, uncertain landscape are sure to raise eyebrows. Here is where comments from readers would be most helpful.
References:
- Curran L.K. et al. Pediatrics 120, 1386-1392 (2007) PubMed
- Fyffe S.L. et al. Neuron 59, 947-958 (2008) PubMed
- Kinney D.K. et al. J. Autism. Dev. Disord. 38, 481-488 (2008) PubMed
- McClellan J.M. et al. JAMA 296, 582-584 (2006) PubMed
- Mehler M.F. and Purpura D.P. Brain Res. Rev. 59, 388-392 (2009) PubMed
- Williams R.S. et al. Arch. Neurol. 37, 749-753 (1980) PubMed
- Aston-Jones G. and Cohen J.D. Annu. Rev. Neurosci. 28, 403-450 (2005) PubMed
- Clayton E.C. et al. J. Neurosci. 24, 9914-9920 (2004) PubMed
- Guy J. et al. Science 315, 1143-1147 (2007) PubMed